Stepping Mechanism of Molecular Motor Protein
Overview
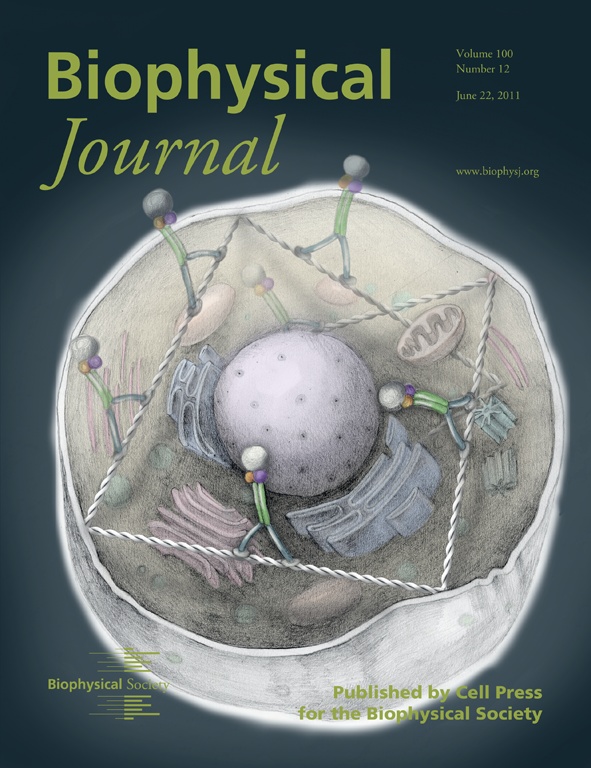
Cells are often compared to busy metropolitan cities, and in this analogy, motor proteins are the delivery trucks running on the roadways and transporting goods to different part of the cell. Motor proteins are found in almost all eukaryotic cells, and they convert chemical energy using ATP hydrolysis into mechanical work that powers their movements along cytoskeletal tracks. Three classes of motor protein superfamily have been characterized: myosin, kinesin, and dynein. While kinesin and dynein move along the microtubule network, myosin moves along actin filaments. Over the years, the structural basis of how motor proteins work has been established by solving the x-ray and cryo-EM structure for different domains of several motor proteins. What remains missing is the dynamic process connecting different states of a motor protein's catalytic cycle. By teaming up with experts of single-molecule techniques from the Selvin lab here at UIUC, we hope to gain a molecular understanding of the stepping mechanism of motor proteins.
Myosin VI
A unique member of the myosin superfamily is myosin VI. Not only does myosin VI "walk" towards the opposite direction along the actin filaments compared to most other myosins, but it also walks with a large step size (~36 nm) using a short lever arm. While the reverse directionality has been attributed to myosin VI's unusual converter subdomain with a unique insert, its large step size cannot be fully explained by the so-called swinging lever arm hypothesis, which states that the length of the lever arm determines the step size of a motor protein.
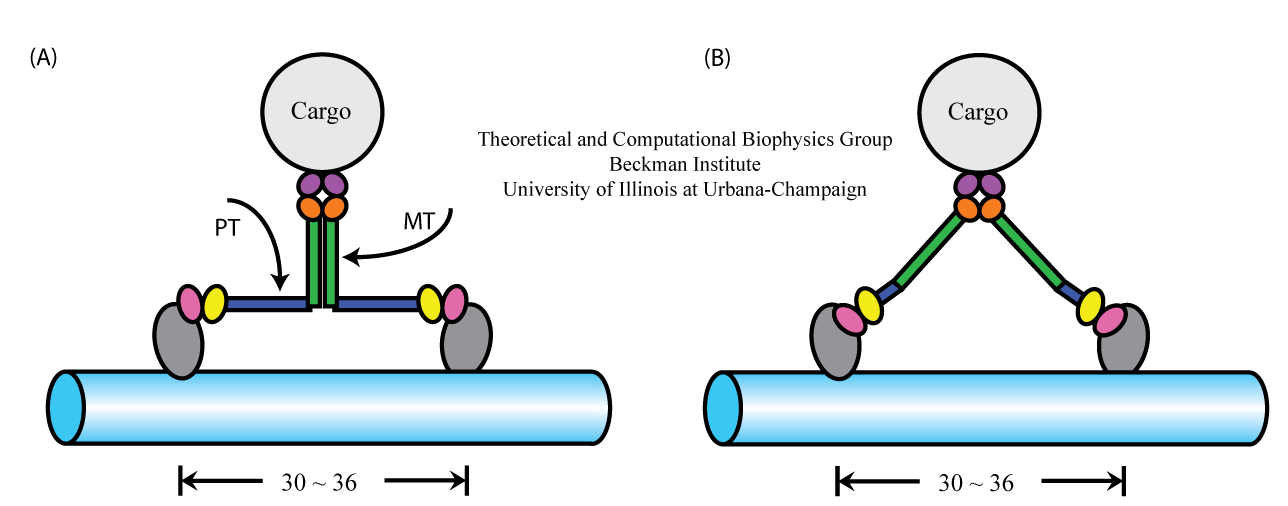
There are currently two models explaining how the myosin VI structure permits a 36-nm step size (Figure 1). The models make contrary assumptions about the complex formation and the effective extension of myosin VI's proximal tail domain. Model A suggests that the medial tail (MT) domains of the myosin VI form a dimer complex, while proximal tail (PT) domain telescopes out from a short (4 nm) three α-helix bundle to a 12-nm length. In model B, the MT domains remain separated and, hence, contribute to the step size, while the PT domain is in its compact, 4-nm long, conformation. To test the validity of these models, in particular the model shown in Figure 1A, we employ experimental means performed by the Selvin lab, as well as various molecular dynamics methods developed in our group. In the following, we addressed two specific questions: (1) whether or not the MT domains dimerization occurs, and (2) whether or not the PT domain can extend from a 4-nm to a 12-nm length.
Dimerization of the myosin VI MT domain
The most prominent property of the MT domain is its repeated pattern of four positive residues followed by four negative residues, called the ER/K motif, and a few hydrophobic residues near the N-terminal end. Our MD simulation results suggest that the positive and negative residues from different monomers can interact to form a stable homodimer. Residue-based coarse-grained (RBCG) model representation was employed to reach the microsecond timescale needed for the self-assembly process of myosin VI. Spontaneous self-association of the MT domain was observed in a 1.2 μs RBCG simulation, as shown in Figure 2 and Movie 1. Molecular Dynamics Flexible fitting (MDFF) was used to reverse the coarse-grained model into full atomic resolution. The dimer is seen to be stable in a subsequent 50 ns all-atom MD simulation (Figure 2 and Movie 2).
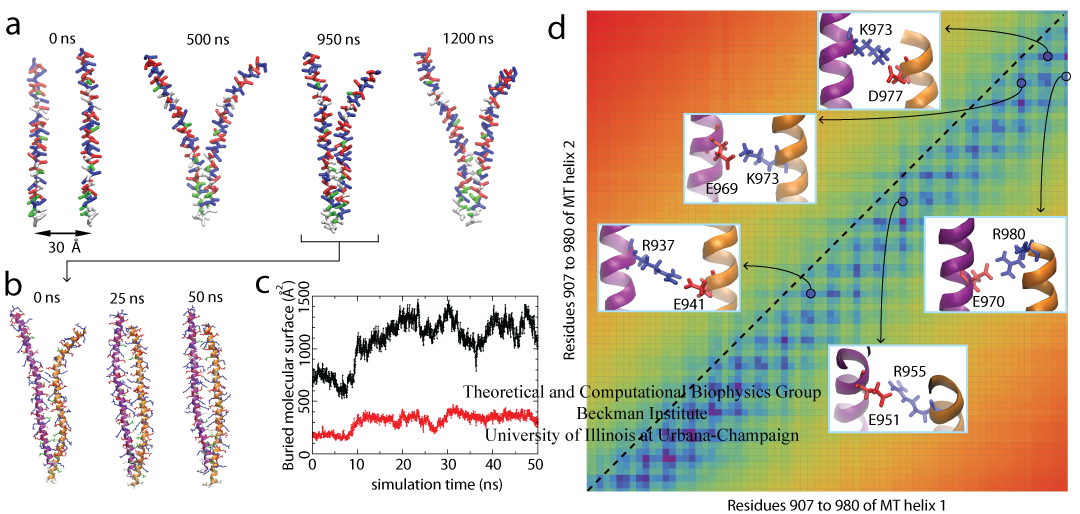
We found that the two identical monomers must assume a spatial offset with respect to each other, such that the positive residues on one monomer can interact with the negative residues on the other monomer. In agreement with the simulation, experimental data also attribute a key role of Coulomb attraction between residues of opposite charge participating in myosin VI dimerization. It is observed, furthermore, that mutations of hydrophobic residues on the MT domain do not abolish myosin VI dimerization, suggesting that Coulomb attraction in the form of salt bridges is the major contribution to dimerization. This conclusion is also supported by calculations of dimerization free energy.
Extension of the myosin VI PT domain
It has been shown by our MD simulations and confirmed by single-molecule experiment that the MT domain of myosin VI is the dimerization region. This observation leads to the conclusion that the PT domain has to unfold to account for the large step size of myosin VI. Subsequent simulations indeed showed that the PT domain of myosin VI readily unfolded, extending from a three-helix bundle to a single helical conformation. Such structural transition tripled the length of the PT domain; thereby the PT domain can act as a lever arm extension to accommodate a step size of 30-36 nm. The two-step unfolding pathway of the myosin VI PT domain is shown in Figure 3(A). A first step involves dissociation of Helix II and III from helix I; a second step involves loss of contact between helix II and III.
The importance of the calmodulins
Calmodulin (short for CALcium MODULated proteIN) is a calcium-binding protein that binds to and regulates a number of different protein. Myosin VI is one of its targets. Calmoduins bind to Myosin VI through the IQ motif on the lever arm and mechanically strengthen the lever arm to act as a rigid leg when myosin VI is walking along actin filaments. We found that the IQ motif bound calmodulin also interacts with the PT domain. Such interactions play a key role during the PT domain extension process. They are not only maintaining the helical structure of the PT domain, but also altering the strain path in myosin VI when external force applied, such as the one originated from MT domain dimerization. Without the IQ motif and its bound calmodulin, the helical structure of the PT domain would be disrupted during the extension process, as shown in Figure 3 (B).
However, the extended PT domain may not be rigid enough to be a mechanical leg. Calmodulins come to the rescue, ... again! Based on sequence analysis, several consensus calmodulin-binding sequences are identified in the PT domain. These potential calmodulin-binding sites open up when the PT domain unfolds from a three α-helical bundle to an extended conformation. Subsequent calmodulin-binding may render the extended PT domain rigid enough to function as a lever arm extension.
In summary, the investigation on MT domain dimerization and PT domain extension established a "walking" model for myosin VI, as shown in Figure 1 (A). It explained how myosin VI realized a large step size with short legs.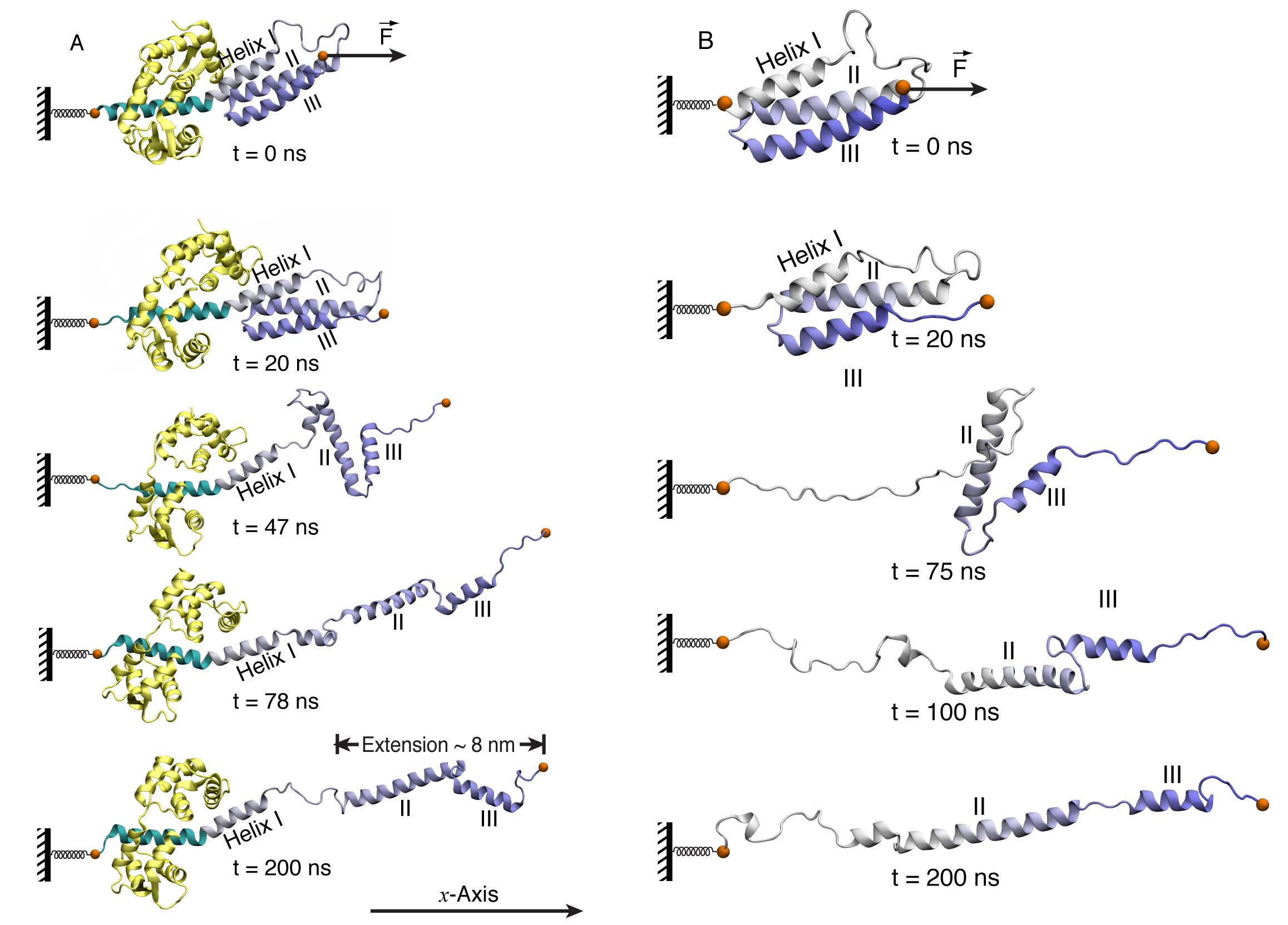
Publications
-
Publications Database Formation of salt bridges mediates internal dimerization of myosin VI medial tail domain. HyeongJun Kim, Jen Hsin, Yanxin Liu, Paul R. Selvin, and Klaus Schulten. Structure, 18:1443-1449, 2010. -
Publications Database Extension of a three-helix bundle domain of myosin VI and key role of calmodulins. Yanxin Liu, Jen Hsin, HyeongJun Kim, Paul R Selvin, and Klaus Schulten. Biophysical Journal, 100:2964-2973, 2011.
Investigators
Collaborators
Page created and maintained by Yanxin Liu.
Last updated: June 8, 2011.